In February the transport minister Jo Johnson called on the rail industry to provide a vision of how it will decarbonise. He stated that he wished to see “all diesel-only trains off the track by 2040” and viewed “alternative-fuel trains powered entirely by hydrogen” as a prize on the horizon. But, seven months previously, transport secretary Chris Grayling announced the cancellation of three electrification schemes, necessitating extra diesel trains. The week beforehand, Grayling stated that the government intended to halt petrol and diesel car production by 2040.
Notwithstanding these apparent contradictions, Johnson is right to stress the need to decarbonise the rail industry. Although trains offer great environmental benefits, the industry cannot rest on its laurels.
Traction power requirements
A train’s power ranges from 450kW for a two-coach local train to 6MW for a 125mph 11-coach electric inter-city train. The respective power-to-weight ratios of these trains are 5.7 and 10.5kW per tonne. Commuter trains do not necessarily travel at high speed but require high power for the acceleration needed to operate a passenger service with frequent stops to an acceptable timetable. An electric multiple unit (EMU) typically has twice the acceleration of a diesel multiple unit.
A UK diesel freight locomotive of typically 2,500kW might haul a train of 2,000 tonnes. Freight services, and depots, also require shunting locomotives to marshal trains. These need a high tractive effort to move heavy loads but only operate at low speeds so require a low-powered engine of, say, 250kW.
As of March 2017, Britain had 14,000 rail passenger vehicles, of which 72% were electric trains and the rest self-powered. There are also 800 freight locomotives, of which 16% are electric. In addition there are 180 shunters.
Hydrogen power
Hydrogen is the most abundant element in the universe but is so chemically active that it does not occur naturally and must be produced. Almost all hydrogen production uses reforming to extract it from organic feedstock, usually methane, which produces CO2.
Electrolysis uses a DC current to split water into hydrogen and oxygen. This can cost 50% more than steam reforming but is a zero-carbon process if the electricity used is generated by renewables. Fuel cells reverse this process, using a catalyst to combine hydrogen with oxygen in the air. This produces electricity and water, the only waste product
Hydrogen is therefore an energy vector. Like electricity it is a man-made form of energy that can be transported and converted into other forms of energy. However, unlike electricity, hydrogen’s energy can be stored on a large scale at relatively low cost. On the Orkney island of Eday, a 0.5MW electrolysis plant exports surplus wind power as hydrogen to Kirkwall on Orkney’s mainland where it powers ferries berthed overnight.
However, this is an inefficient process. Typical efficiencies for electrolysis and fuel cells are respectively 68% and 52%. Compressing hydrogen for storage, typically at 350bar, requires 6% of its chemical energy. So the overall cycle efficiency is 33%. Hence hydrogen traction requires 3kW of electricity to deliver 1kW of power to the wheel. An electric train has no on-board energy conversion so only needs 1.2kW.
This low overall cycle efficiency potentially undermines the green credentials of hydrogen trains as they require 2½ times the electrical energy of a comparable electric train, especially if hydrogen is delivered by the much cheaper CO2-producing reforming process. However, if otherwise surplus overnight wind-turbine generating capacity is used to produce and store hydrogen, this low efficiency is not an issue. Used in this way, hydrogen production also addresses intermittency issues associated with electrical generation from renewables.
A further constraint is hydrogen’s low energy density. At 350bar, the volumetric energy density of hydrogen is 4.6MJ/litre, compared with 35.8MJ/litre for diesel. So a hydrogen train requires fuel storage eight times the size of a diesel train’s fuel tank.
Falling costs
Hydrogen is becoming big business, as shown by the launch of the Hydrogen Council at Davos in January 2017 when 13 transport and energy companies pledged to invest €10bn in hydrogen technologies over the next five years. This is the result of the worldwide interest in hydrogen as an alternative fuel for cars, buses, standby supplies and other uses. UK examples are Aberdeen’s fleet of 10 hydrogen buses, hydrogen cars for the Metropolitan Police, and the Scottish government’s plan to purchase a hydrogen-powered ferry for the Orkney Isles in 2021.
Whatever the rail industry does, worldwide production of hydrogen for transport is expected to increase to the extent that, by the mid-2020s, it will exceed that required for industrial processes. As hydrogen becomes more readily available and its supply infrastructure expands, costs are likely to reduce, making it an increasingly attractive fuel for rail vehicles.
There have certainly been significant improvements in fuel-cell technology since NASA used it on its lunar landing programme when the electricity produced cost £1,000 per kilowatt. In the 10 years since 2001, one manufacturer had increased the output of its fuel cells from 25 to 33kW while reducing the volume from 365 to 125 litres and increasing efficiency from around 42% to 52%.
With the restricted space available on a train for its traction equipment, improvements such as these now make hydrogen a viable rail traction technology.
Hydrogen trains
The University of Birmingham produced Britain’s first hydrogen-powered locomotive in 2012 to compete in the IMechE’s Railway Challenge. This was a miniature locomotive with a 1.1kW fuel cell supplemented with batteries to give a total power output of 4.4kW. Four years later, Alstom unveiled the world’s first passenger-carrying hydrogen-powered train, the two-car iLint, which has 138 seats plus capacity for 190 standing passengers.
The iLint is a hybrid unit with a 200kW fuel cell and 225kW lithium-ion traction battery that stores surplus fuel-cell energy and recovers braking energy. This is managed by iLint’s traction control system which ensures that the fuel cell operates at optimum performance and delivers a peak power-to-weight ratio of 8kW/tonne. On each coach are fuel cells and roof tanks that store 99kg of hydrogen at 350bar which the unit consumes at the rate of around 0.3kg/km, giving a range of 600km at speeds of up to 140km/h. It takes 15 minutes to refuel.
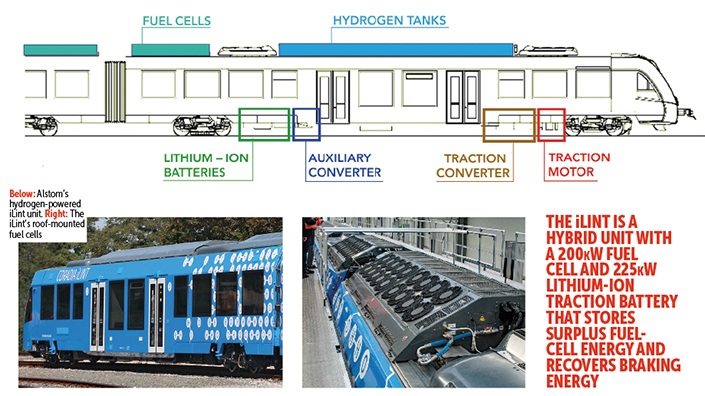
It is no coincidence that the first customer for these trains, the German state of Lower Saxony, is the country’s leading wind-power state with an installed turbine capacity of more than 9,000MW. In 2017, the state signed contracts for 14 iLints which are to enter service on the 240km Cuxhaven-to-Buxtehude line from 2021. Until then, the two prototypes will operate on these lines. Other German states have signed letters of intent for 50 iLint units.
In Britain, Alstom announced in May its plans to convert an unspecified number of class 321 EMUs to hydrogen operation. These are expected to enter service in 2021-22.
Battery trains
Battery-powered trains also offer zero emissions at the point of use but have limited range owing to the low battery energy density – 0.56MJ/litre for lead acid and 2.63MJ/litre for lithium-ion. And batteries cannot be instantly refuelled.
Battery trains have potential for use on short non-electrified branches off an electrified line as was demonstrated in 2015 when a four-car EMU with a 7.2-tonne, 424kWh battery pack operated services on the Harwich branch. This showed that, when charged for 70% of its run under electric power, the battery could deliver an EMU-comparable performance for 77km.
Powering a train beyond electrified wires for much longer distances would require the development of batteries with much higher energy density. It would be wrong to base transport policy on such an unpredictable development.
The electric solution
With electric trains comprising 72% of the UK passenger fleet, options to decarbonise rail traction must consider how their electricity is generated. The Netherlands has significantly reduced the CO2 footprint of its trains as the state railway has a contract with its energy provider specifying that all electric trains will run on sustainable energy.
Currently 42% of the UK rail network is electrified. This compares with 76% in the Netherlands, 71% in Italy and 61% in Spain. The key question is whether the UK electrified network should be further expanded to include intensively used routes and those used by freight trains. One reason for doing so is that, with many new rail vehicles on order, there will soon be many surplus EMUs, owing to the cancelled electrification schemes, which would otherwise be scrapped.
Electric trains offer lower fuel and maintenance costs of the order of millions of pounds per vehicle over its lifetime. For example, diesel fuel accounts for 40% of Virgin West Coast’s traction cost, despite only 15% of its fleet being diesel powered. One reason for this is that electric traction can feed braking energy back into the electrical distribution system. High-speed or heavy self-powered trains can’t reuse this energy as they cannot store the huge amounts generated. Electric trains are also more than twice as reliable as diesel trains.
The partners on a 2015 trial of a battery-powered unit
A key benefit of electric trains is that they are more powerful than self-powered trains owing to the limited space on a diesel train for fuel, power unit and its cooling group. The maximum power of an electric train is determined by the current that can be drawn from the 25,000V overhead wires. This is around 7.5MW which is more than that required for current UK trains.
Bi-mode trains that can operate in both diesel and electric mode have been used to justify electrification cutbacks. Yet when in diesel mode these trains operate at only two thirds of their electric power. A nine-car bi-mode train has electric traction power of 4.5MW, while its five diesel engines deliver 3.5MW, of which about 3MW is available to power the train.
Although a recent study concluded that it would be feasible to use hydrogen trains instead of electrifying the Toronto Metrolinx commuter network, a close study of this report reveals hydrogen’s limitations for high-powered rail vehicles. Metrolinx services use 12-coach double-decker trains which the study proposes should be hauled by two 5,600kW hydrogen-powered locomotives – that’s 13 times the power output of a single iLint coach.
These locomotives would thus contain 13 times the volume of the iLint’s fuel cells, hydrogen tanks and traction batteries for which a two-vehicle locomotive would be required. Thus, if hydrogen powered, Toronto’s trains would require four vehicles to haul them and carry their hydrogen.
Furthermore this study did not consider the 33% overall cycle efficiency of hydrogen traction. This has significant implications for the required power generation and hydrogen supply infrastructure.
No one solution
Electrification is potentially a zero-carbon option for intensively used railways and offers many other benefits. It is currently the only practical way of replacing diesel freight locomotives, although shunting locos could be battery or hydrogen powered. Electrification does have a high capital cost. However, recent electrification projects have cost at least twice the amount they should (see “Getting electrification right,” Rail Engineer June 2018).
Hydrogen offers great potential for less busy routes but is unlikely ever to be an appropriate solution for high-speed, intensive commuter or freight services. Battery-powered vehicles are also feasible for branch lines off electrified routes.
The question of how to decarbonise rail has no single answer, but electrification must be the main solution.
'There are challenges, but hydrogen trains can be viable'
“Hydrogen offers us an opportunity to reduce emissions and air pollution from diesel trains,” says Dr Jenifer Baxter, head of engineering at the Institution and author of a forthcoming report on hydrogen trains which is due to be published by the IMechE in November. “There are a number of challenges that would be faced in developing a UK-wide network of hydrogen trains, such as storage, transportation and refuelling networks,” says Baxter. “However, that does not mean hydrogen trains are not viable.”
QUESTIONS TO ANSWER
“Where would the hydrogen for these trains be produced? How will the carbon dioxide emissions be managed? Can they be collected and moved to storage? When considering the storage of hydrogen on a refuelling network, this has to be available at strategic points across the network that enable easy refuelling in addition to storage on the train for use.”
TRANSPORTATION
”If hydrogen is not produced at a refuelling site then it has to be transported there. There are options for this: hydrogen can be moved in compressed-gas cylinders or cryogenic tankers; there is also potential to use hydrogen pipelines and possibly in the future hydrogen could be transported using a repurposed gas distribution grid that we currently use for natural gas.”
HYDROGEN CLUSTERS
“The overall efficiency of a hydrogen train is about a third that of an electric train, so on an intensively used railway it may be better to feed electricity directly into a train instead of using it to create hydrogen. But, creating hydrogen clusters around industry where hydrogen is produced could be a solution for localised transport systems. For example, trains and buses operating near industries where hydrogen is produced could use hydrogen as a fuel, as production, storage and refuelling would be nearby.”
Content published by Professional Engineering does not necessarily represent the views of the Institution of Mechanical Engineers.