Your knees carry you through life. But at some point after 50 years of use they will begin to creak and get stiff. It’s no wonder – the joints go through between one and two million cycles every year. And once your knees start creaking the prognosis is not good.
The cartilage that covers the surface of joints and allows bones to slide over one another may be damaged. Cartilage helps to reduce friction, prevent damage and support your weight when you move, bend and stretch.
Cartilage can be repaired but the options are limited. Surgeons can try to encourage new cells to grow in the affected area or add healthy tissue from other parts of the body, with or without success. In the worst cases a knee replacement is needed, but this typically only lasts for 15 to 20 years.
Still, more than 70,000 knee replacements take place in England and Wales every year, and the number is on the up. Surgeons in the US predict that demand for knee replacements will increase by 500% over the next two decades. So the need to find better ways to repair cartilage has never been greater.
Medical engineers have grappled for years with the challenges of producing materials that can mimic cartilage. Any artificial cartilage that they produce must be mechanically strong, durable, lubricated and compatible with living tissue.
A new wave of biomaterials and manufacturing techniques that promise to meet these needs are in the pipeline. Companies and research groups are looking at rapid prototyping, CAD and 3D printing to create implantable scaffolds that will allow healthy cells to regrow.
One of these groups is led by Professor James Yoo at the Wake Forest Institute for Regenerative Medicine in North Carolina. Yoo and his colleagues have developed a method of printing artificial cartilage. The system integrates an electrospinning machine, which creates tiny fibre scaffolds of a synthetic polymer, with an inkjet printer that lays down cells.
Previously it had not been possible to combine natural and synthetic biomaterials using the same machine. “The major advantage of our technique is that we are using inkjet printing to precisely control deposition of cells in complex patterns within the layers,” says Yoo.
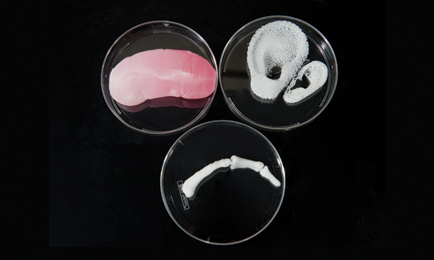
These tiny implantable scaffolds are made by 3D printing
The researchers created tiny devices and tested them in mice for between two and eight weeks. Upon inspection, the constructs appeared to develop the structures and properties typical of elastic cartilage.
The ultimate goal of the project is to develop an implant that can replace damaged cartilage. In practice, MRI scans of a patient’s knee could be used as a blueprint to guide the printing of a matching construct. Yoo says that the technique is many years away from use in patients but is a “promising new technology” that could simplify the manufacture of complicated tissues. Since producing the cartilage, the group has gone on to develop a more sophisticated robotic 3D system to print muscle, cartilage and kidney cells.
In the UK, a company called Orthox is using a similar rapid prototyping approach to create artificial cartilage implants that can replace the meniscus of the knee. The implants are made of a protein called fibroin, which is extracted from silk. Orthox chief executive Nick Skaer says: “Silk is one of the strongest materials known to man. It can absorb a huge amount of energy before it breaks.”
Fibroin is the long structural component of silk fibres and is similar in structure to a protein abundant in cartilage. Two scientists in the zoology department at the University of Oxford who worked on spider silk saw the appeal of the material and spun out a company to try to exploit it in 2002.
Their firm, Spinox, focused on spinning silk to generate resilience and strength. Six years later Orthox was formed to develop orthopaedic devices from the silk protein. Over the past four years Skaer and his colleagues have been working to produce a method to do this, funded by a £1.6 million grant from the Wellcome Trust.
A patented process extracts the protein from raw silk fibres without degrading the molecules. First the silk fibres are cleaned to remove surface contaminants. Next the hydrogen bonds that hold the silk fibroin molecules together are disrupted without breaking the covalent bonds that hold the backbone of the molecule together.
This creates a viscous solution of fibroin which is injected into a generic mould produced from CAD files generated from MRI scans of knee joints. The solution is then processed using the same ionic reagents, temperatures and pressures that spiders use to spin silk fibres. The resulting devices have a high degree of molecular alignment, which allows hydrogen bonds to reform between and within the molecules. “It’s this bonding that is crucial to the performance of the device,” says Skaer.
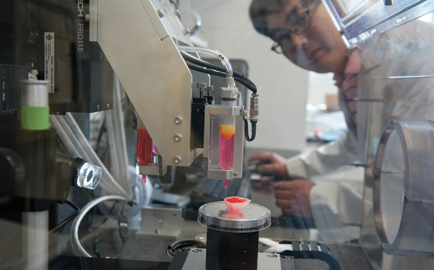
Making body parts: Integrated 3D printing
The devices are 85% porous. “It’s like a very strong resilient sponge,” explains Skaer. Once implanted into a patient, the porosity allows cells from the surrounding tissue to grow into the device and deposit cells to help keep it in place. Without this, the device could fall out of the joint once the initial sutures have worn out. The device has a smooth surface to make sure that there is no wear to the opposing tissues in the joint.
Dr Leigh Fleming is part of a team at the Centre for Innovative Manufacturing in Advanced Metrology at the University of Huddersfield that is inspecting the smoothness of the implant’s surface. “It’s just like looking at the surface finish of a bearing in an aerospace component where you need very smooth surfaces for low wear rate and improved function,” she explains. One day she is working on the fan blades for massive jet engines, the next she is scrutinising the surface nanostructure of tiny bits of artificial cartilage.
She says that using surface metrology on this type of device is innovative. Fleming measures the surface characteristics using various techniques. One is optical interferometry, which uses the interference of scanning white light beams to accurately measure the various heights across the surface.
She says: “That gives us nanometer-scale resolution so we can see if there are any peaks that might be worn away during use.” The instrument splits a beam of light into two and shines one of them onto a sample of the material, which is then reflected back up into the optical path. The second beam is reflected into a reference mirror. The texture of the scanned surface can be recreated digitally by comparing the reflection of the two beams.
Other tests include atomic force microscopy, which moves a very low load tapping stylus across the surface to build up height data, and scanning electron microscopy to look at the material’s ultrastructure.
In the longer term Orthox wants to make higher-value cartilage replacement devices that are patient specific. A project funded by the Technology Strategy Board will look at developing the technology to create these, using data from routine MRI joint scans taken before surgery. The money will also be used to put the meniscal cartilage product through clinical trials.
Once the firm has tackled the knee, it hopes to adapt the technology for other medical uses, including nerve and gastrointestinal repair and wound closure.
Seaweed may aid knee repair
Materials and mechanical scientists at the Harvard School of Engineering and Applied Sciences in Massachusetts have developed a stretchy and tough gel that could one day be used to replace worn cartilage.
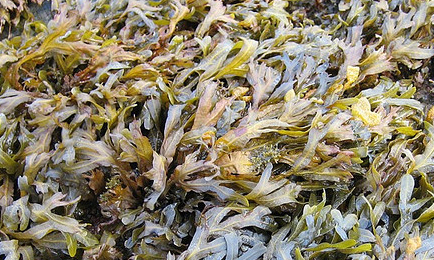
The hydrogel combines polyacrylamide and alginate, a seaweed extract often used to thicken food, at an 8:1 ratio. The polymers form a complex network of cross-linked chains that allow the molecules to pull apart slightly over a large area, rather than cracking.