In the early 2000s a biomedical engineer named Professor Richard Kitney was asked by the chairman of a Royal Academy of Engineering committee what he thought would have the largest impact on engineering in the 21st century. When he answered, the others in the room laughed. Most of them had replied digital communication.
Kitney’s response was “systems biology,” and he’s since had the last laugh. A decade on, he and his colleague Professor Paul Freemount have become the UK’s pioneers of an emerging field of science known as synthetic biology which promises to revolutionise industry.
Put simply, synthetic biology is the engineering of biology – where scientists and engineers design and build biological systems to perform specific functions. Using chemical techniques, researchers create sequences of synthetic DNA which are then inserted into bacterial cells, re-programming them to produce drugs, fuels and plastics or suck up carbon dioxide, among other things.
Work around DNA has been going on for many years. Genetic engineering, for example, which involves the insertion of one or two new genes into a cell, has been possible since the 1970s.
But new, fast DNA sequencing techniques born out of the Human Genome Project have now made it possible for synthetic biology to be used to design and produce a cell’s entire genome from scratch.
Synthetic biology also differs from genetic engineering in that it places the emphasis on developing platforms and technologies that make the job more reliable and straightforward.
In fact, the discipline relies on three principal tenets of engineering: modularisation, characterisation and standardisation. Researchers break down a biological system into a series of components, such as genes and proteins, and each of these parts is characterised. This enables researchers to observe how different components will behave and function in certain contexts, and to design systems appropriately. The whole process is standardised, so that systems can be built from well-defined modules.
Essentially, it is similar to designing an electronic circuit, as standard components are arranged in a certain way to achieve a specific result. But using biological building blocks instead of silicon ones.
“This has the potential to have a major economic impact,” explains Kitney. He and many others believe that taking a synthetic approach to biology could transform the field just as it did chemistry in the mid-to-late 19th century, when chemists realised that instead of merely analysing existing molecules they could synthesise new ones. This gave rise to the chemical and pharmaceutical industries that we know today. Kitney says: “Synthetic biology will perform a similar role in the 21st century.”
He adds: “With synthetic biology you can produce new industrial processes and new industries. This is likely to be a major growth area.”
The government is also counting on synthetic biology delivering economic growth. In 2008 Kitney and Freemount were awarded £8 million by the Engineering and Physical Sciences Research Council (EPSRC) to set up a national Centre for Synthetic Biology and Innovation based in the faculty of engineering at Imperial College, London. Earlier this year, the EPSRC doled out a further £5 million to be shared by five universities to develop platform technologies that will speed up the commercialisation of the science.
When announcing the recent funding, David Willetts, minister of state for universities and science, said that synthetic biology could provide solutions to many of humanity’s “most pressing issues”.
He added: “This investment will lay the groundwork for the commercialisation of research, ensuring academics and industry can realise the full potential of this exciting area of science.”
But UK investment pales in comparison with that seen recently in the US, where in excess of $550 million has been pumped into the field from public and private sources. This includes a $500 million project led by BP and the US Department of Energy to create an Energy Biosciences Institute, where synthetic biology will play a key role.
Using synthetic biology to create the next generation of biofuels is on the agenda at Kitney’s laboratory in London, too. His team is working on ways to programme the DNA of bugs to feed on landfill waste, process it and convert it into fuel.
Kitney explains: “You can design different types of bugs to eat paper, plastics or wood and produce fuels like ethanol and benzene that fit into current refinery processes.”
The processes used to create these so-called advanced biofuels would be far more efficient than many currently in use. In Brazil, for example, a well-established method creates ethanol biofuel from sugar cane. But about 90% of the sugar cane biomass is wasted, as it cannot be turned into ethanol.
Kitney is also working on producing biological computers – cells that can perform like mini microprocessors. By inserting modified DNA into Escherichia coli cells, he and his colleagues have created stable logic gates which process data by switching on and off.
The next stage of their research is to produce biological adders – simple digital devices that can do calculations and potential stepping stones on the way to creating biological microprocessors.
In the future, biological microprocessors could be used to deliver drugs to the parts of the body that need them most. A tiny unit that combines biosensors with a biological microprocessor and a spherical nanocage of proteins that open and release anti-cancer drugs once activated by a cancer cell could offer targeted treatments.
To make such types of technology possible will require input from engineers, biologists, chemists, mathematicians and physicists, and there are now several interdisciplinary centres hosting such research in the UK.
At the University of Cambridge, Dr Jim Haselhoff is working on a proposal to build on the institution’s tradition of integrating physical sciences into biology. His proposal, iLab, will give physical scientists better access to the latest biological techniques.
Haselhoff’s work looks at how shapes are built into biological systems. He says that biological systems develop differently from conventionally engineered systems. In biology there are no blueprints and components are not built one at a time. Instead, a biological system starts with one cell that divides, then the cells differentiate, creating order as they go. These systems interact, are self-repairing and highly complex.
He adds: “Its quite different from conventional engineering, but very exciting as a general theme of how engineering systems might work.”
But manipulating biology to create forms of life that do not exist in nature is controversial. Critics condemned the work of US researcher Craig Venter, who announced in 2009 that he had created the world’s first synthetic life form. Religious groups questioned whether Venter was playing God.
Kitney says there is a “strong movement” in UK universities to take into account the social, ethical and environmental considerations of work from the outset. Haselhoff adds that social scientists are involved in all the foundational research initiatives that he is aware of.
He says: “As our entire human culture is based on non-sustainable practices, we need to address the possibility of finding replacements.”
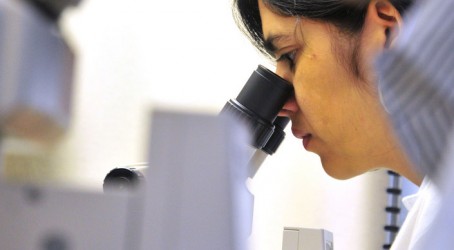
Undergraduate blockbusters
Students are given the chance to engineer biology in the annual International Genetically Engineered Machine (iGEM) competition. The competition, which started at the Massachusetts Institute of Technology in 2003, sees teams of undergraduates from universities worldwide compete by using synthetic biology techniques to design and build a biological system that can operate in a living cell to solve a specific problem.
Teams have access to an online library of standardised biological parts, known as BioBricks, which they can use to create their entry. Each BioBrick is a sequence of
DNA that is known to have a specific function.
As part of the competition teams must create new BioBricks to add to the library by characterising new sections of DNA relevant to their projects in the laboratory.
A team from University College London recently made it through the European heats in the Netherlands to win a place in the final to be held in the US in November.
The team is working to engineer marine bacteria to aggregate the tiny particles of plastic that pollute the North Pacific.